Highlights:
Issue 4 - Apr 2025
Issue 4 Article 4
The Elixir of Immortality
25/4/20
By:
Ong Peng Ce Linus
Edited:
Elijah Chew Ze Feng
Tag:
Molecular Biology and Biochemistry
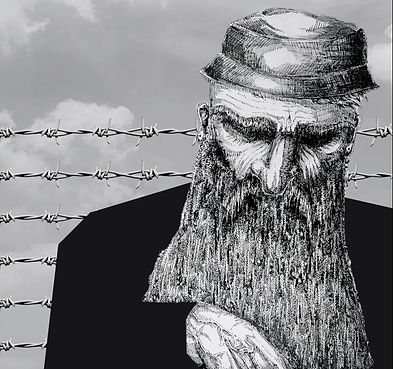
All animals live long, but some animals live longer than others.
While ageing and death are often noted as the ubiquitous end of all life, nature’s bizarre quirks have led to species that seem to hijack this process and simply not get older. By identifying and understanding the mechanisms underlying this, scientists hope to one day slow down and even reverse ageing in humans as we know it, and thus to try and extend the human lifespan.
What is ageing?
While ageing is often colloquially referred to as the act of “getting older”, one way that biologists define it is the decline of the eight hallmarks of health. These eight hallmarks, as set out by López-Otín and Kroemer (2020), are the integrity of barriers, containment of perturbations, recycling and turnover, integration of circuitries, rhythmic oscillations, homeostatic resilience, hormetic regulation, and repair and regeneration. As a result of the deterioration of these hallmarks, individuals gradually begin to lose physiological integrity, which affects normal, healthy functioning and increases the likelihood of death.
How then does ageing lead to a decline in the hallmarks of health? Building upon their landmark 2013 paper, López-Otín et al. set out in 2023 twelve key hallmarks of ageing, on which scientists have focused their research efforts. These hallmarks generally fall into three broad categories.
First, there are the primary hallmarks, or in other words, the direct causes of ageing. These include: genomic instability, which refers to an increase in mutations in an organism’s genome as it replicates; telomere shortening, which refers to the shortening of the repeated protective TTAGGG sequences at the end of chromosomes, which help to maintain chromosomal integrity and prevent DNA strands from binding to one another; epigenetic alterations, which refers to changes in the non-genomic factors that regulate gene expression; loss of proteostasis, which refers to the loss of control of protein translation and protein levels due to oxidative stress and other factors; and macroautophagy, which refers to the intentional “self-eating” and destruction of organelles by a cell.
Second, there are antagonistic hallmarks, or, damage control factors. These include: deregulated nutrient sensing, which refers to a loss of control of the response to nutrients like glucose; mitochondrial dysfunction, which refers to a decline in the normal function of the “powerhouse of the cell”; and cellular senescence, which refers to an increase in the proportion of cells that enter a state of non-cell division.
Third, there are integrative hallmarks, which then directly lead to the phenotype. These include: stem cell exhaustion, which refers to a general decrease in the activity of stem cells, which are cells with the potential for further differentiation into other cell types; altered intercellular communication, which refers to changes in the inter-cellular signalling molecules produced by cells for tissue coordination; chronic inflammation, which while normally is used to recruit immune cells and signal damage, leads to a gradual wearing out of normal and immune cells; and dysbiosis, which refers to changes in an organism’s microbiome.
How can we hijack ageing?
While scientists have been working hard to use these hallmarks to hopefully slow or reverse ageing, nature has offered several examples of organisms that seem to buck the trend. Enter the ‘immortal’ jellyfish, Turritopsis dohrnii. How does it do it? It seems that these small and transparent animals can turn back time by reverting to an earlier stage of their life cycle under certain conditions.
Normally, the life cycle of a jellyfish begins with a fertilised egg that grows into a planula (the larval stage). This planula then attaches to a surface and grows into a polyp, which resembles a tube with a mouth on one end and a foot at the other. This initial polyp then grows a colony of other polyps that later form an outgrowth or a stack of separate segments that break away from the colony. This then leads to the next stages of the life cycle: the ephyra stage (when it is a small jellyfish) and then the medusa stage (when it is a fully-formed adult).
Interestingly, under environmental stress like starvation and injury, Turritopsis dohrnii is capable of reverting to the polyp phase. This thus bypasses all the associated problems of ageing in the medusa stage. Ignoring the problem of Theseus' Ship, this means that as long as the whole organism is not consumed or killed in any other way, it can live forever.
Another animal with a claim to fame, though not to immortality, is the simple Hydra. Often found in freshwater ponds, the Hydra is a distant-but-not-too-distant relative of jellyfish as both belong to the phylum Cnidaria. While they cannot cycle back and forth through their life cycles like jellyfish, they do not undergo the ageing hallmark of cellular senescence, due to the FoxO genes that enable their cells to keep dividing. While found in many animals, including humans, Hydra appears to have an overexpression of these genes that somehow helps them to keep senescence out.
Moreover, while not immortal, lobsters address one of the hallmarks of ageing by preventing telomere shortening. Through high expression of telomerase, the enzyme that helps to maintain telomeres at the end of chromosomes, lobsters can theoretically maintain their chromosomes and continuously divide. However, moulting, which is an energetically expensive process, often limits a lobster’s natural lifespan and denies them immortality.
And, last on our list, the Arctica islandica (otherwise known as the Ocean Quahog Clam) is capable of astronomically long lifespans. In fact, the longest recorded living specimen was 507 years old, and likely only died because researchers had to freeze it to accurately determine its age. The clam’s claim to immortality is likely due to a reduced hallmark of ageing: genomic instability. Due to a notably low production of reactive oxygen species (think of the free radicals that health supplements claim to prevent), it appears that Arctica islandica does not have high buildups of them, which thus reduces damage to DNA, RNA, and proteins.
Why can’t we live forever?
Despite all the inspiration to be found in nature, it has been difficult to apply what we can learn from other species to humans. For one, evolutionary differences sometimes make it impossible to replicate what can be found in nature (imagine trying to mimic the Turritopsis dohrnii by reverting to a fetus). Moreover, even when there are overlaps (such as in the presence of a shared gene), the greater complexity of the human genome and epigenetic regulation pathways often make it difficult to see reproducible results.
Thus, while much research and funding has been focused on fighting ageing for quite some time, meaningful results may only come after our lifetimes. And, even when therapies and solutions do arrive, costs and inequities may keep them out of reach from many of us and in the hands of a rich and select few.
References
Berthold, E. (2021, May 24). The animals that can live forever. Curious. https://www.science.org.au/curious/earth-environment/animals-can-live-forever
Frater, J. (2019, May 26). 10 Ageless animals that do not grow old. Listverse. https://listverse.com/2019/05/28/10-ageless-animals-that-do-not-grow-old/
López-Otín, C., Blasco, M. A., Partridge, L., Serrano, M., & Kroemer, G. (2013). The hallmarks of aging. Cell, 153(6), 1194–1217. https://doi.org/10.1016/j.cell.2013.05.039
López-Otín, C., Blasco, M. A., Partridge, L., Serrano, M., & Kroemer, G. (2023). Hallmarks of aging: An expanding universe. Cell, 186(2), 243–278. https://doi.org/10.1016/j.cell.2022.11.001
López-Otín, C., & Kroemer, G. (2020). Hallmarks of health. Cell, 184(1), 33–63. https://doi.org/10.1016/j.cell.2020.11.034
Latest News